Chemically Modified Cyclodextrins
Many different chemical moieties may be introduced into the Cyclodextrin molecule by reaction with the hydroxyl groups lining the upper and lower ridges of the toroid; for example, methyl, hydroxypropyl, carboxymethyl, and acetyl.
Since each Cyclodextrin hydroxyl group differs in its chemical reactivity, the reaction process produces an amorphous mixture of thousands of positional and optical isomers. Any collection of hydroxypropyl-b-cyclodextrin molecules is a highly complex mixture of various isomeric forms of variously substituted b-cyclodextrin derivatives. There are 221-1 or 2,097,151 possible geometric isomers for hydroxypropyl-b-cyclodextrin (see figure 2). 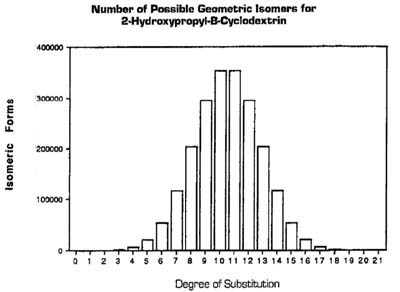
Number of Possible Geometric Isomers for 2-Hydroxypropyl B Cyclodextrins In the range of 3-11, each integral degree of substitution is related to various possibilities described by the term: a!/b! ----- (a-b)! where "a" is the number of hydroxyl groups in b-cyclodextrin and "b" is the degree of substitution. For the trisubstituted material, for example, there are 1330 possible positional isomers. For a mixture containing 3-11 degrees of substitution there are 1,401,059 possible positional isomers. Each of these isomers is then associated with 2(n) optical isomers and since each beta cyclodextrin nucleus contains 28 chiral centers and each hydroxypropyl-b-cyclodextrin adds an additional asymmetric carbon, the number of possible isomers is truly astronomical. These calculations do not take into consideration hydroxypropyl polymers attached to the Cyclodextrin nucleus. In this sense, the mixture studied here is similar to hydroxyethyl cellulose, hydroxypropyl cellulose and hydroxypropyl methyl cellulose, all of which are pharmaceutically acceptable excipients. The task of separating each component of this mixture is the same as if one were asked to separate the isomers of hydroxypropyl cellulose; it is simply impossible. If one attempted to produce the "unitary" compounds chemically, a similarly difficult experience would be expected since even when specific stoichiometries are used, widely disparate mixtures of hydroxypropyl-b-cyclodextrin are obtained upon reaction of propylene oxide and b-cyclodextrin. This property of amorphousness is important to certain physico-chemical properties of the chemically modified Cyclodextrins (CMCD) and has beneficial effects on aqueous solubility and toxicity. The aggregate substitution that takes place is described by a term called the degree of substitution (DS); for example, a hydroxypropyl beta Cyclodextrin (HPBCD) with a DS of 5.0 would be composed of a distribution of isomers of HPBCD in which the average number of hydroxypropyl groups per HPBCD molecule was five. Degree of substitution is usually determined by mass spectrometry (MS) or nuclear magnetic resonance (NMR) spectroscopy and does not give information as to the exact location of the hydroxypropyl substituents (C6, C2, C3) or the distribution of those substituents around the Cyclodextrin molecule (mono, di, tri, poly). Theoretically the maximum degree of substitution is 18 for a-Cyclodextrin, 21 for b-Cyclodextrin, and 24 for g-Cyclodextrin; however; substituents with their own hydroxyl groups present opportunities for additional hydroxylations. One may use DS to calculate the average molecular weight of the Cyclodextrin derivative. This randomness of position and type of substitution causes the resultant Cyclodextrin product to be amorphous, which contributes to its greatly enhanced aqueous solubility compared to the crystalline parent. It may be possible to optimize certain physical properties of aqueous solutions of specific Cyclodextrin derivatives by controlling DS; such properties as surface activity have been shown by Yoshida and Müller *[10-11] to vary with DS. These investigators have also measured the change in water content of the powder as a function of DS. Irie et al *[12] further looked at the actual distribution of substituents around the cyclodextrin molecule. The implication of these studies is that biological effects (for example, hemolysis) of given Cyclodextrin derivatives in solution may be eliminated or minimized; other parameters that may be optimized include viscosity, storage conditions and tabletting properties. Evidence to date does not provide a meaningful correlation between the degree of substitution and biological effects. It is likely that such effects may be observable only with great differences in DS, for example 3 and 11 or 5 and 18, rather than between 6 and 9 or 2 and 5 or 11 and 13. Chemical modification of the Cyclodextrins can also change the phase solubility characteristics of the complexes formed *[13]; that is, precipitation of the complex with the addition of more Cyclodextrin (B type solubility) can be changed to continued solubilization with the addition of Cyclodextrin (A type solubility). Finally, chemical modification of Cyclodextrins can result in unexpected changes in solubility behavior, as illustrated by the property of dimethyl-Beta-Cyclodextrin to precipitate from aqueous solution as the temperature is raised above 55° C. The point to be made here is that derivatives of Cyclodextrins provide the innovative formulation scientist with rational options for optimizing formulations by specific drug, by concentration of drug, by storage and manufacturing requirements, and by route of administration. Table 2 lists some of the CMCD's as well as the natural Cyclodextrins that are available from CTD, Inc. The first CMCD to receive regulatory approval for use in humans will become the flagship product of a new drug delivery industry - probably HPBCD.
Natural Cyclodextrins | Modified natural Cyclodextrins | Chemically modified Cyclodextrins |
---|
Alpha-Cyclodextrin (TACyclodextrin) | glucosyl-alpha-Cyclodextrin (TG1A) | 2-hydroxypropyl-beta-Cyclodextrin (THPB) | Beta-Cyclodextrin (TBCyclodextrin) | maltosyl-alpha-Cyclodextrin (TG2A) | 2-hydroxypropyl-gamma-Cyclodextrin (THPG) | Gamma-Cyclodextrin (TGCyclodextrin) | glucosyl-beta-Cyclodextrin (TG1B) | hydroxyethyl-beta-Cyclodextrin (THEB) |
| maltosyl-beta-Cyclodextrin (TG2B) | methyl-beta-Cyclodextrin (TRMB) | * parentheses contain catalogue number designation of these products available from CTD, Inc. Gainesville, Florida as TRAPPSOL® cyclodextrins. | Table 2 |
"Chemically Modified" Cyclodextrins
Formula | Description | Molec. Wt. Adduct | Cat # | R',R'' | = | -H | "Natural" Cyclodextrins [alpha, beta, gamma] |
| TACD TBCD TGCD | R',R'' | = | -CH3 | Methyl Cyclodextrin's | 15 | TMB | R',R'' | = | -CHOH-CH3 | Hydroxyethyl Cyclodextrin's | 45 | THEB | R',R'' | = | -CH2 CHOH-CH3 | 2-Hydroxypropyl Cyclodextrin's | 59 | THPB THPG | R'[α,1 -6] | = | -C6H11O5 | Glucosyl Cyclodextrin's | 163 | TG1A TG1B | R'[α1 -6] | = | -C12H21O10 | Maltosyl Cyclodextrin's | 325 | TG2A TG2B TG2BM | R'=a substituent on a primary carbon atom R''=a subsituent on a secondary carbon atom | Table 3
Solubility Table for Methyl Beta
·
Water at 25°C 80g/100ml
·
Chloroform at 25° C 70g/100ml
·
Methanol at 25°C 90g/100ml
·
Tetrahydrofuran at 25° 65g/100ml
Theoretically a cyclodextrin molecule (α, β, or γ) can have up to 3 (n) substituents, where n is the number of glucopyranose units which comprise the CD molecule. This is referred to as the degree of substitution (DS). The DS refers to substituents other than hydrogen; substituents may be all of one kind or mixed. Fractional degrees of substitution are also possible as all of the CD molecules do not necessarily undergo substitution to the same extent, and a weighted average is used.
|